A neutrino is a subatomic particle that is very similar to an electron,
but has no electrical charge and a very small mass, which might even be
zero. Neutrinos are one of the most abundant particles in the universe.
Because they have very little interaction with matter, however, they are
incredibly difficult to detect. Nuclear forces treat electrons and
neutrinos identically; neither participate in the strong nuclear force,
but both participate equally in the weak nuclear force. Particles with
this property are termed leptons. In addition to the electron (and it’s anti-particle, the positron),
the charged leptons include the muon (with a mass 200 times greater than
that of the electron), the tau (with mass 3,500 times greater than that
of the electron) and their anti-particles.
Both the muon and the tau, like the electron, have accompanying
neutrinos, which are called the muon-neutrino and tau-neutrino. The
three neutrino types appear to be distinct: For instance, when
muon-neutrinos interact with a target, they will always produce muons,
and never taus or electrons. In particle interactions, although
electrons and electron-neutrinos can be created and destroyed, the sum
of the number of electrons and electron-neutrinos is conserved. This fact leads to dividing the leptons into three families, each with a charged lepton and its accompanying neutrino.
To detect neutrinos, very large and very sensitive detectors are
required. Typically, a low-energy neutrino will travel through many
light-years of normal matter before interacting with anything.
Consequently, all terrestrial neutrino experiments rely on measuring the
tiny fraction of neutrinos that interact in reasonably sized detectors.
For example, in the Sudbury Neutrino Observatory, a 1000 ton heavy
water solar-neutrino detector picks up about 1012 neutrinos each second. About 30 neutrinos per day are detected. Wolfgang Pauli first postulated the existance of the neutrino in
1930. At that time, a problem arose because it seemed that both energy
and angular momentum were not conserved in beta-decay. But Pauli pointed
out that if a non-interacting, neutral particle–a neutrino–were
emitted, one could recover the conservation laws. The first detection of
neutrinos did not occur until 1955, when Clyde Cowan and Frederick
Reines recorded anti-neutrinos emitted by a nuclear reactor.
Natural sources of neutrinos include the radioactive decay of
primordial elements within the earth, which generate a large flux of
low-energy electron-anti-neutrinos. Calculations show that about 2
percent of the sun’s energy is carried away by neutrinos produced in
fusion reactions there. Supernovae too are predominantly a neutrino
phenomenon, because neutrinos are the only particles that can penetrate
the very dense material produced in a collapsing star; only a small
fraction of the available energy is converted to light. It is possible
that a large fraction of the dark matter of the universe consists of
primordial, Big Bang neutrinos.
The fields related to neutrino particles and astrophysics are
rich, diverse and developing rapidly. So it is impossible to try to
summarize all of the activities in the field in a short note. That said,
current questions attracting a large amount of experimental and
theoretical effort include the following: What are the masses of the
various neutrinos? How do they affect Big Bang cosmology? Do neutrinos
oscillate? Or can neutrinos of one type change into another type as they
travel through matter and space? Are neutrinos fundamentally distinct
from their anti-particles? How do stars collapse and form supernovae?
What is the role of the neutrino in cosmology?
One long-standing issue of particular interest is the so-called
solar neutrino problem. This name refers to the fact that several
terrestrial experiments, spanning the past three decades, have
consistently observed fewer solar neutrinos than would be necessary to
produce the energy emitted from the sun. One possible solution is that
neutrinos oscillate–that is, the electron neutrinos created in the sun
change into muon- or tau-neutrinos as they travel to the earth. Because
it is much more difficult to measure low-energy muon- or tau-neutrinos,
this sort of conversion would explain why we have not observed the
correct number of neutrinos on Earth. Source : Scientific Americn
Ovidio Universe: Neutrino
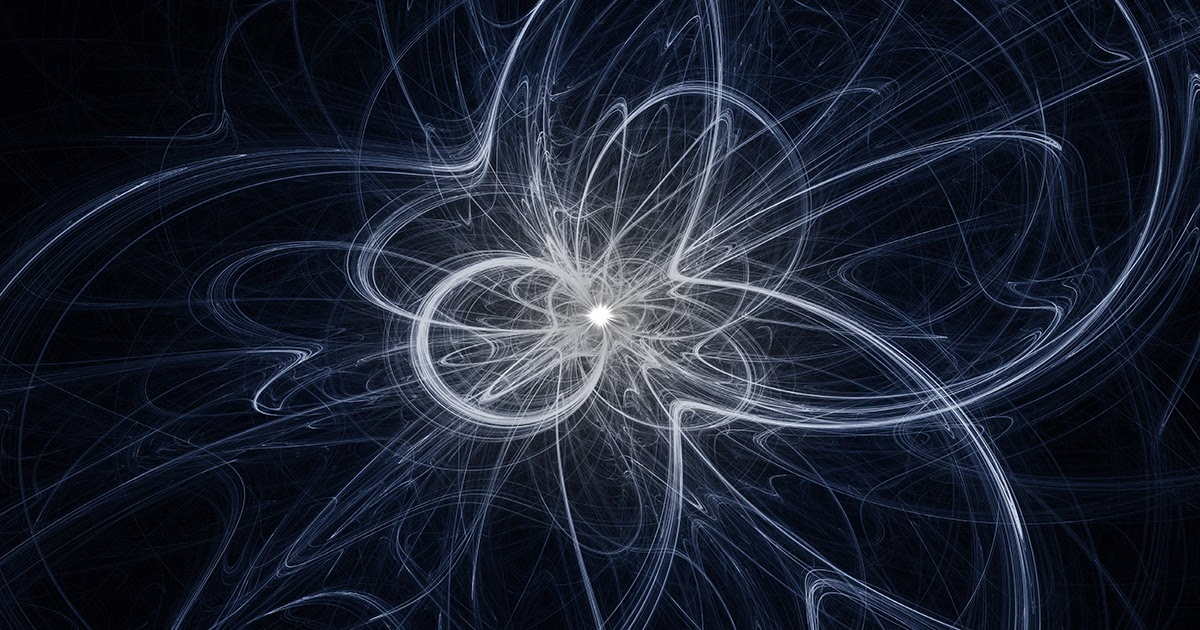